Frozen records to help guide climate change adaptation in the tropical Andes
- laearlycareer
- Feb 7, 2022
- 4 min read
Author: Filipe Lindau
At the top of the highest mountains in Bolivia and Peru we find more than 90% of the Earth’s tropical glaciers (Kaser, 1999). They exist at these low latitudes mainly due to two factors: altitude and precipitation. In northeastern Bolivian Cordillera, where the mountains reach altitudes above 6,000 m above the sea level (asl), and moisture arrives from the Amazon basin in considerable amounts during the austral summer (Garreaud et al., 2003), snow remains on the top of these high mountains throughout the year (Figure 1). In this Bolivian region there is a net accumulation of snow above altitudes around 5,500 m asl, so the glaciers are sustained (Rabatel et al., 2013). The role of precipitation in the occurrence of glaciers becomes clear if we travel to southwestern Bolivia, where even areas 6,000 m above sea level can be free of glaciers due to aridity.

Figure 1: The Cordillera Real in the Bolivian Andes. Photo: F. Lindau.
In recent decades, glaciers in the tropical Andes have retreated in close relationship to the observed increase in high-elevation surface air temperature (Rabatel et al., 2013). There are several temperature increase scenarios that project the near future of these glaciers, however, there are still many uncertainties when considering changes in precipitation, as well as other factors that influence the mass and energy balance of these glaciers (Vuille et al., 2018). Many concerns are associated with retreating glaciers, including water supply for agriculture and human consumption, as well as concerns related to ecosystem integrity and glacial hazards. Thus, a detailed understanding of glaciological, hydrological and climatic aspects is necessary to design effective adaptation measures for glacial retreat.
Massive amounts of water vapor are evapotranspired to the atmosphere by the Amazon rainforest, which are condensed and evapotranspired several times throughout the Amazon basin and supply the tropical Andes with moist air and subsequent snowfall. Deforestation of the low-lying Amazon threatens the recycling of precipitation and the flux of water vapor exported to the Andes, which would favor an even greater glacial retreat. However, observations of precipitation and atmospheric circulation in the Amazon and Andean regions are recent and sparse to provide accurate projections for future precipitation on tropical Andes glaciers. Thus, more observational and modeling analyzes are needed to improve our understanding of the connections between Amazonian forests and the climate and water cycle in the Andes (Espinoza et al., 2020).
Motivated by this challenge, an ice core was drilled in Nevado Illimani by a French, Russian, Bolivian and Brazilian team to interpret their records in relation to atmospheric circulation and precipitation (Figure 2). Ice cores are recovered in the glacier accumulation zone, where successive snow deposits form thick layers of ice, and several characteristics of the atmosphere are maintained at the time of snow precipitation. The atmospheric dust signature in the ice core has been interpreted as a proxy for turbulent conditions that are caused by local and regional convective activity (Lindau et al., 2021), which in turn accounts for most of the precipitation in the tropical Andes (Garreaud et al., 2003). The strong winds caused by convective activity are able to suspend giant dust particles (greater than 20 µm in diameter) in the vicinity of Nevado Illimani and then print an ice core signal (Lindau et al., 2021).
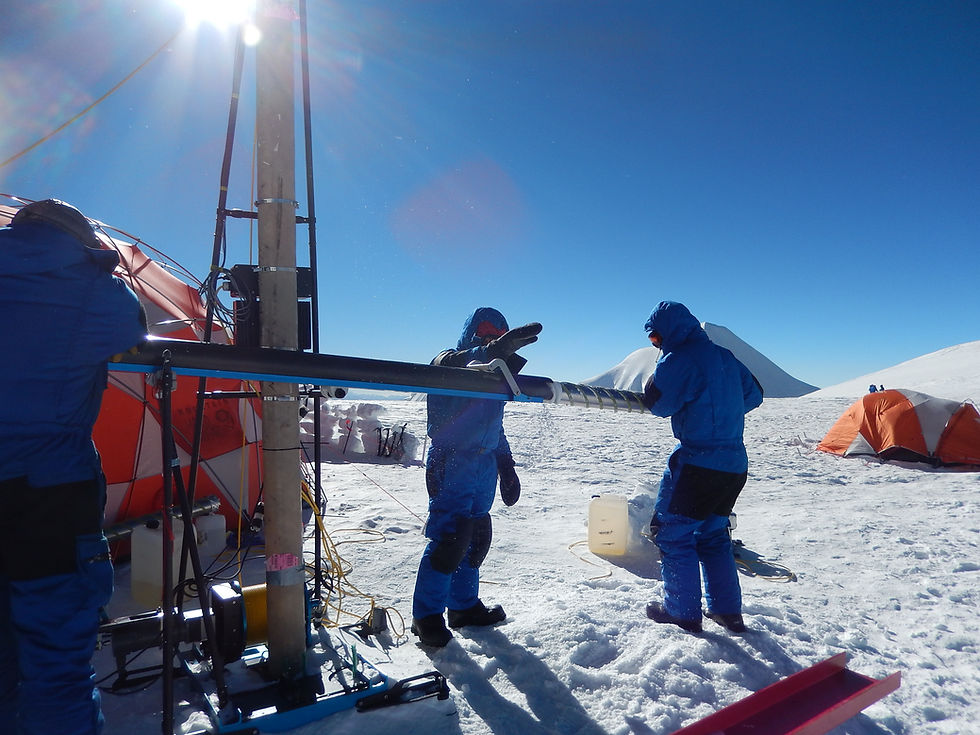
Figure 2: Drilling an ice core during the Ice Memory campaign in 2017 at 6,350 m altitude in Nevado Illimani, Bolivia.
Photo: F. Lindau.
Therefore, Andean ice core records can provide valuable information about the flux of water vapor between the Amazon basin and the tropical Andes. Furthermore, a greater understanding of this issue could be a key factor in combating deforestation in the Amazon and mitigating the retreat of glaciers in the tropical Andes. References:
Espinoza JC, Garreaud R, Poveda G, Arias PA, Molina-Carpio J, Masiokas M, Viale M, Scaff L. 2020. Hydroclimate of the Andes Part I: Main Climatic Features. Front Earth Sci 8: 1–20. doi: 10.3389/feart.2020.00064 Garreaud R, Vuille M, Clement AC. 2003. The climate of the Altiplano: Observed current conditions and mechanisms of past changes. Palaeogeogr Palaeoclimatol Palaeoecol 194(1–3): 5–22. doi: 10.1016/S0031-0182(03)00269-4 Kaser G. 1999. A review of the modern fluctuations of tropical glaciers. Glob Planet Change 22(1–4): 93–103. doi: 10.1016/S0921-8181(99)00028-4 Lindau FGL, Simões JC, Delmonte B, Ginot P, Baccolo G, Paleari CI, Di Stefano E, Korotkikh E, Introne D, Maggi V, et al. 2021. Giant dust particles at Nevado Illimani: a proxy of summertime deep convection over the Bolivian Altiplano. Cryosph 15(3): 1383–1397. doi: 10.5194/tc-15-1383-2021 Rabatel A, Francou B, Soruco A, Gomez J, Cáceres B, Ceballos JL, Basantes R, Vuille M, Sicart JE, Huggel C, et al. 2013. Current state of glaciers in the tropical Andes: A multi-century perspective on glacier evolution and climate change. Cryosph 7(1): 81–102. doi: 10.5194/tc-7-81-2013 Vuille M, Carey M, Huggel C, Buytaert W, Rabatel A, Jacobsen D, Soruco A, Villacis M, Yarleque C, Elison Timm O, et al. 2018. Rapid decline of snow and ice in the tropical Andes – Impacts, uncertainties and challenges ahead. Earth-Science Rev 176(November 2016): 195–213. Elsevier. doi: 10.1016/j.earscirev.2017.09.019
Comments